Flustra foliacea and other hydroid/bryozoan turf species on slightly scoured circalittoral rock or mixed substrata
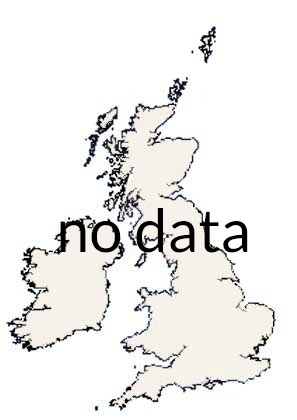
Researched by | Dr Harvey Tyler-Walters | Refereed by | This information is not refereed |
---|
Summary
UK and Ireland classification
Description
A widespread biotope which has been split into several related entities. The biotope is characterized by silt- and scour-tolerant species which occur in varying proportions around the country, but Flustra foliacea tends to dominate. This biotope is characteristic of silty rocky habitats, tending to be moderately exposed to wave action and with a moderate tidal flow which create the slight scour conditions (compared to silted rocky habitats in sheltered conditions). The species associated with and therefore characterizing the different Flustra biotopes vary from region to region, ranging from the relatively low species-rich MCR.Flu.Flu found on North Sea coasts to the similar but far richer biotopes with sponges and hydroids on the west of Britain and Irish Sea coasts (MCR.Flu.HByS). There are also several other related biotopes: these include the Urticina (MCR.Urt.Urt) and Ciocalypta (MCR.Urt.Cio) biotopes which occur at rock-sediment interfaces; ascidian-dominated biotopes with Flustra (MCR.StoPaur) and several other biotopes characterized by other slight scour-tolerant or turbid-water species such as Sabellaria spinulosa which include Flustra (MCR.Sspi and MCR.MolPol.Sab) and Alcyonidium diaphanum (MCR.SNemAdia). Only use this biotope if records do not fit into other categories. (Information taken from the Marine Biotope Classification for Britain and Ireland, Version 97.06: Connor et al., 1997a, b).
Depth range
-
Additional information
None
Listed By
Habitat review
Ecology
Ecological and functional relationships
This biotope is dominated by sessile, permanently fixed, suspension feeding invertebrates that are, therefore, dependant on water flow to provide: an adequate supply of food and nutrients; gaseous exchange; remove metabolic waste products; prevent accumulation of sediment, and disperse gametes or larvae. The majority of species found in this biotope are adapted to strong water flow, siltation and a degree of sediment scour. Little is known of ecological relationships in circalittoral faunal turf habitats (Hartnoll, 1998) and the following has been inferred from studies of other epifaunal communities (Sebens, 1985; 1986).
- Few plants are found in this biotope but include encrusting coralline algae and occasionally small red algae (Sebens, 1985; Hartnoll, 1998).
- Suspension feeders on bacteria, phytoplankton and organic particulates and detritus include sponges (Polymastia spp. and Esperiopsis fucorum), soft corals and anemones( e.g. Alcyonium digitatum and Metridium senile), erect and encrusting bryozoans (e.g. Flustra foliacea, and Bugula spp.), brittlestars (e.g. Ophiothrix fragilis), barnacles (e.g. Balanus balanus), caprellid amphipods, porcelain crabs (e.g. Pisidia longicornis), polychaetes (e.g. Sabella pavonina and Spirobranchus spp.) and sea squirts (e.g. Clavelina lepadiformis). However, the water currents they generate are probably localized , so that they are still dependent on water flow to supply adequate food.
- Passive carnivores of zooplankton and other small animals include, hydroids (e.g. Tubularia indivisa and Nemertesia antennina), soft corals (e.g. Alcyonium digitatum), while larger prey are taken by Urticina felina and Metridium senile (Hartnoll, 1998).
- Sea urchins (e.g. Echinus esculentus and Psammechinus miliaris) are generalist grazers, removing ascidians, hydroids and bryozoans and potentially removing all epifauna, leaving only encrusting corallines and bedrock. Sea urchins were shown to have an important structuring effect on the community and epifaunal community succession (Sebens, 1985; 1986; Hartnoll, 1998).
- Other grazers include top shells (e.g. Jujubinus miliaris) and Calliostoma zizyphinum, which grazes hydroids, and small crustaceans (e.g. amphipods).
- Specialist predators of hydroids and bryozoans include the nudibranchs (e.g. Antiopella cristata, Doto spp. and Onchidoris spp.) and pycnogonids, (e.g. Achelia echinata), while the nudibranch Tritonia hombergi preys on Alcyonium digitatum, and some polychaetes take hydroids.
- Starfish (e.g. Asterias rubens and Crossaster papposus) are generalist predators feeding on most epifauna, including ascidians.
- Scavengers include polychaetes, small crustaceans such as amphipods, starfish and larger decapods such as hermit crabs (e.g. Pagurus bernhardus) and crabs (e.g. Hyas coarctatus).
- Mobile fish predators are likely to include gobies (e.g. Pomatoschistus spp.), butterfish (Pholis gunnellus), wrasse and eelpout (Zoarces viviparus) feeding mainly on small crustaceans, while species such as flounder (Platichthys flesus) are generalists feeding on ascidians, bryozoans, polychaetes and crustaceans (Sebens, 1985; Hartnoll, 1998)
Competition
Intra and interspecific competition occurs for food and space. Filter feeders reduce the concentration of suspended particulates and deplete food to other colonies/individuals downstream (intra and inter specific competition). Sebens (1985, 1986) demonstrated a successional hierarchy, in which larger, massive, thick growing species (e.g. large anemones, soft corals and colonial ascidians) grew over low lying, or encrusting growth forms such as halichondrine sponges, bryozoans, hydroids and encrusting corallines. The epifauna of vertical rock walls became dominated by large massive species, depending on the degree of predation, especially by sea urchins. However, encrusting bryozoans and encrusting corallines may survive overgrowth (Gordon, 1972; Sebens, 1985; Todd & Turner, 1988). In this biotope the degree of sediment scour and siltation probably exerts a controlling factor on the succession (see temporal change below) and is dominated by species tolerant of sediment scour and high water flow.
Seasonal and longer term change
No information on seasonal or temporal change in Flustra dominated communities was found and the following information has been inferred from available studies of subtidal epifaunal communities (Sebens, 1985, 1986; Hartnoll, 1983, 1998).
Seasonal changes
Some species such as the ascidians Ciona intestinalis and Clavelina lepadiformis are effectively annual while some hydroids an bryozoans, may show annual phases of growth and dormancy or regression. For example, Flustra foliacea becomes dormant in winter, Bugula species die back in winter to dormant holdfasts, while the uprights of Nemertesia antennina die back after 4-5 month and exhibit three generations per year (spring, summer and winter) (see MarLIN reviews; Hughes, 1977; Hayward & Ryland, 1998; Hartnoll, 1998).
Succession
Sebens (1985, 1986) described successional community states in the epifauna of vertical rock walls. Clear space was initially colonized by encrusting corallines, rapidly followed by bryozoans, hydroids, amphipods and tube worm mats, halichondrine sponges, small ascidians (e.g. Dendrodoa carnea and Molgula manhattensis), becoming dominated by the ascidian Aplidium spp., or Metridium senile or Alcyonium digitatum. High levels of sea urchin predation resulted in removal of the majority of the epifauna leaving encrusting coralline dominated rock. Reduced predation allowed the dominant epifaunal communities to develop, although periodic mortality (through predation or disease) of the dominant species resulted in mixed assemblages or a transition to another assemblage (Sebens, 1985, 1986). Sea urchin predation may play a significant role in freeing space for colonization in this community. Succession will be dependant on species tolerance to silt and sediment scour. For example, the sub-biotope MCR.Flu.Flu is relatively species poor due to high silt levels, while more sponges, ascidians, bryozoans and hydroids occur in increased scour but reduced silt habitats (e.g. MCR.Flu.Hocu or MCR.Flu.Hbys).
Community stability
Long-term studies of fixed quadrats in epifaunal communities demonstrated that while seasonal and annual changes occurred, subtidal faunal turf communities were relatively stable, becoming more stable with increasing depth and substratum stability (i.e. bedrock and large boulders rather than small rocks) (Osman, 1977; Hartnoll, 1998). Many of the faunal turf are long-lived, e.g. 6 -12 years in Flustra foliacea, 5-8 years in Ascidia mentula, over 20 years in Alcyonium digitatum, 8-16 years in Echinus esculentus and probably many hydroids (Stebbing, 1971a; Gili & Hughes, 1995; Hartnoll, 1998).
Habitat structure and complexity
- The bedrock is covered by a layer of encrusting corallines, and encrusting bryozoans, overgrown by dominant erect bryozoans and hydroids (e.g. Flustra foliacea, Bugula species, Nemertesia antennina, Thuiaria thuja) interspersed with encrusting sponges (e.g. Polymastia spp.), ascidians (e.g. Dendrodoa grossularia), Alcyonium digitatum and Urticina felina. The dominance by Flustra foliacea and other erect bryozoans and hydroids and ascidians forms a faunal turf over the substratum.
- The faunal turf provides interstices and refuges for a variety of small organisms such as nemerteans, polychaetes, and amphipods, while the erect species provide substrata for caprellid amphipods, which use them as 'platforms' to suspension feed.
- The erect bryozoans and hydroids support a variety of epizoics that use them as substratum and in some cases affect their growth rates. For example, Flustra foliacea supported 25 species of bryozoan, 5 hydroid species, some sessile polychaetes, barnacles, lamellibranchs and tunicates (Stebbing, 1971b). The bryozoans Bugulina flabellata, Crisia spp. and Scrupocellaria spp. were major epizoics. Scrupocellaria spp. settled preferentially on the youngest, distal, portions of the frond, possibly to elevate their branches into faster flowing water (Stebbing, 1971b). Similarly, Alcyonidium parasiticum is epizoic on hydroid stems or the bryozoan Cellaria spp. and the sponge Esperiopsis fucorum may grow on the stem of Tubularia species or on the test of ascidians.
- Mobile species include decapods crustaceans such as shrimp, crabs and lobsters, sea urchins, starfish and fish.
- Gobies, shannies and butterfish probably utilize available rock ledges and crevices, while large species such as flounder and cod probably feed over a wide area.
- Pockets of sediment that accumulate between boulders or in crevices (where present) may support benthic infaunal species such as Mya truncata and Sabella pavonina.
- The rock may support the rock boring bivalve Hiatella arctica.
- The biotope may show spatial variation in community complexity and exhibit a mosaic of different species patches (Hartnoll, 1998), due to colonization of areas recently cleared by predation, disease or physical disturbance in the process of re-colonization. The upper edges or boulders or rocky outcrops, most directly in water flow, tend to exhibit the most species rich and abundance faunal turfs, while species richness decreases with proximity to the sediment/ rock interface, which favours species such as the sponges Polymastia spp. or the anemone Urticina felina. Areas subject to increased scour or vertical surfaces tend to be dominated by tube worms such as Spirobranchus triqueter (Stebbing, 1971b, Eggleston, 1972b; Sebens, 1985, 1986; Connor et al., 1997a; Brazier et al., 1998; Hartnoll, 1998).
- Periodic disturbance of the community due to physical disturbance by storms, extreme scour, or fluctuations in predation, especially by sea urchins, may encourage species richness by preventing dominance by a few species (Osman, 1977; Sebens, 1985, 1986; Hartnoll, 1998).
Productivity
Circalittoral faunal turf biotopes are primarily secondary producers. Food in the form of phytoplankton, zooplankton and organic particulates from the water column together with detritus and abraded macroalgal particulates from shallow water ecosystems are supplied by water currents and converted into faunal biomass. Their secondary production supplies higher trophic levels such as mobile predators (e.g. fish) and scavengers (e.g. starfish and crabs) and the wider ecosystem in the form of detritus (e.g. dead bodies and faeces). In addition, reproductive products (sperm, eggs, and larvae) also contribute to the zooplankton (Hartnoll, 1998). However, no estimates of faunal turf productivity were found.
Recruitment processes
Most of the species within this biotope produce short-lived, larvae with relatively poor dispersal capacity, resulting in good local recruitment but poor long range dispersal. Although, the biotope occurs within moderately strong to strong water flow that could remove a large proportion of the reproductive output, most reproductive propagules are probably entrained within the reduced flows within the faunal turf or in turbulent eddies produced by flow over the uneven substratum, resulting in turbulent deposition of propagules locally. Many species are capable of asexual propagation and rapidly colonize space. For example:
- Hydroids are often the first organisms to colonize available space in settlement experiments (Gili & Hughes, 1995). The characteristic hydroids in this biotope (e.g. Abietinaria abietina and Sertularia argentea) lack a medusa stage, releasing planula larvae. Planula larvae swim or crawl for short periods (e.g. <24hrs) so that dispersal away from the parent colony is probably very limited (Sommer, 1992; Gili & Hughes, 1995). However, Nemertesia antennina releases planulae on mucus threads, that increase potential dispersal to 5 -50m, depending on currents and turbulence (Hughes, 1977). Most species of hydroid in temperate waters grow rapidly and reproduce in spring and summer. Few species of hydroids have specific substrata requirements and many are generalists. Hydroids are also capable of asexual reproduction and many species produce dormant, resting stages, that are very resistant of environmental perturbation (Gili & Hughes, 1995). Hughes (1977) noted that only a small percentage of the population of Nemertesia antennina in Torbay developed from dormant, regressed hydrorhizae, the majority of the population developing from planulae as three successive generations. Rapid growth, budding and the formation of stolons allows hydroids to colonize space rapidly. Fragmentation may also provide another route for short distance dispersal. Hydroids may potentially disperse over a wide area in the long-term as dormant stages, or reproductive adults, rafting on floating debris or hitch hiking on ships hulls or in ballast water (Cornelius, 1992; Gili & Hughes, 1995).
- The brooded, lecithotrophic coronate larvae of many bryozoans (e.g. Flustra foliacea, Securiflustra securifrons, and Bugula species), have a short pelagic lifetime of several hours to about 12 hours (Ryland, 1976). Flustra foliacea releases larvae in spring (February- April) (Eggleston, 1972a; Hayward & Ryland, 1998), while Bugulina flabellata exhibits two generations per year and releases larvae between April to October (Dyrynda & Ryland, 1982). Recruitment is dependant on the supply of suitable, stable, hard substrata (Eggleston, 1972b; Ryland, 1976; Dyrynda, 1994). In temperate waters most bryozoans species tend to grow rapidly in spring and reproduce maximally in late summer, depending on temperature, day length and the availability of phytoplankton (Ryland, 1970). However, even in the presence of available substratum Ryland (1976) noted that significant recruitment in bryozoans only occurred in the proximity of breeding colonies. For example, Hatcher (1998) reported colonization of slabs, suspended 1 m above the sediment, by Bugula fulva within 363 days while Castric-Fey (1974) noted that Bugulina turbinata, Crisularia plumosa and Bugula calathus did not recruit to settlement plates after ca two years in the subtidal even though present on the surrounding bedrock. Similarly, Keough & Chernoff (1987) noted that Bugula neritina was absent from areas of seagrass bed in Florida even though substantial populations were present <100m away.
- Echinoderms are highly fecund, producing long-lived planktonic larvae with high dispersal potential but recruitment in echinoderms is poorly understood, often sporadic, variable between locations and dependant on environmental conditions such as temperature, water quality and food availability. Recruitment was reported to be sporadic in Echinus esculentus, e.g. Millport populations showed annual recruitment, whereas few recruits were found in Plymouth populations between 1980-1981 (Nichols, 1984). Bishop & Earll (1984) suggested that the population of Echinus esculentus at St Abbs had a high density and recruited regularly whereas the Skomer population was sparse, ageing and had probably not successfully recruited larvae in the previous 6 years. In Ophiothrix fragilis recruitment success is heavily dependent on environmental conditions including temperature and food availability. In years after mild winters Ophiothrix fragilis occurred in extremely high densities in the Oosterschelde estuary in Holland (Smaal, 1994). However, echinoderms such as Echinus esculentus, and Asterias rubens are mobile and widespread and are likely to recruit by migration from other areas.
- Sponges may proliferate both asexually and sexually. A sponge can regenerate from a broken fragment, produce buds either internally or externally or release clusters of cells known as gemmules which develop into a new sponge. Most sponges are hermaphroditic but cross-fertilization normally occurs. There is a mass spawning of gametes through the osculum, which enter a neighbouring individual in the inhalant current. Fertilized eggs may be discharged into the sea where they develop into a planula larva. But in the majority of species development is viviparous, whereby the larva develops within the sponge and is then released. Larvae have a short planktonic life of a few hours to a few weeks, so that dispersal is probably limited and asexual reproduction probably results in clusters of individuals.
- Anthozoans, such as Alcyonium digitatum and Urticina felina are long lived with potentially highly dispersive pelagic larvae and are relatively widespread. They are not restricted to this biotope and would probably be able to recruit rapidly (refer to the Key Information reviews). Similarly, Metridium senile has a long lived, dispersive planktonic planula larva. It is also capable of reproducing asexually by budding from the base, and colonizes space aggressively, forming clumps (Sebens, 1985; Hartnoll, 1998). Juveniles are susceptible to predation by sea urchins or overgrowth by ascidians (Sebens, 1985; 1986).
- Ascidians such as Molgula manhattensis and Clavelina lepadiformis have external fertilization but short lived larvae (swimming for only a few hours), so that dispersal is probably limited (see MarLIN reviews). Where neighbouring populations are present recruitment may be rapid but recruitment from distant populations may take a long time.
- Mobile epifauna will probably recruit from the surrounding area as the community develops and food, niches and refuges become available, either by migration or from planktonic larvae. For example, Hatcher (1998) noted that the number of mobile epifaunal species steady increased over the year following deployment of settlement panels in Poole Harbour.
Time for community to reach maturity
No information was found on the development of this biotope and the following has been inferred from studies of similar epifaunal communities (Sebens, 1985, 1986; Hartnoll, 1998).
The recolonization of epifauna on vertical rock walls was investigated by Sebens (1985, 1986). He reported that rapid colonizers such as encrusting corallines, encrusting bryozoans, amphipods and tubeworms recolonized within 1-4 months. Ascidians such as Dendrodoa carnea, Molgula manhattensis and Aplidium spp. achieved significant cover in less than a year, and, together with Halichondria panicea, reached pre-clearance levels of cover after 2 years. A few individuals of Alcyonium digitatum and Metridium senile colonized within 4 years (Sebens, 1986) and would probably take longer to reach pre-clearance levels.
Jensen et al. (1994) reported the colonization of an artificial reef in Poole Bay, England. They noted that erect bryozoans, including Crisularia plumosa, began to appear within 6 months, reaching a peak in the following summer, 12 months after the reef was constructed. Similarly, ascidians colonized within a few months e.g. Aplidium spp. Sponges were slow to establish with only a few species present within 6-12 months but beginning to increase in number after 2 years, while anemones were very slow to colonize with only isolated specimens present after 2 years (Jensen et al., 1994.). In addition, Hatcher (1998) reported a diverse mobile epifauna after a years deployment of her settlement panels.
Flustra foliacea occurs in this biotope. New colonies of Flustra foliacea take at least 1 year to develop erect growth and 1-2 years to reach maturity, grow slowly (Stebbing, 1971a; Eggleston, 1972a), and would probably several years to reach high abundance, depending on environmental conditions. Recruitment may be enhanced in areas subject to sediment abrasion, where less tolerant species are removed, making more substratum available for colonization, especially if larval release in spring coincides with the end of winter storms. The wreck of a small coaster (the M.V. Robert) off Lundy became dominated by erect bryozoans, including occasional Flustra foliacea, within 4 years of sinking, when it was first surveyed (Hiscock, 1981).
Overall, encrusting bryozoans, hydroids, and ascidians will probably develop a faunal turf within less than 2 years, and Flustra foliacea can evidently colonize and reach an abundance of occasional (1-5% cover) within 4 years. Slow growing species such as Flustra foliacea and some sponges and anemones, will probably take many years to develop significant cover, so that this biotope may take between 5 -10 years to develop an stable community after disturbance, depending on local conditions.
Additional information
None
Preferences & Distribution
Habitat preferences
Depth Range | |
---|---|
Water clarity preferences | |
Limiting Nutrients | |
Salinity preferences | |
Physiographic preferences | |
Biological zone preferences | |
Substratum/habitat preferences | |
Tidal strength preferences | |
Wave exposure preferences | |
Other preferences | Sediment scour |
Additional Information
The distribution map includes records of both the MCR.Flu biotope and its sub-biotopes. Flustra foliacea is associated with strong currents and areas subject to sediment abrasion (Stebbing, 1971a; Knight-Jones & Nelson-Smith, 1977; Hartnoll, 1983; Holme & Wilson, 1985) and requires stable hard substrata (Eggleston, 1972b; Ryland, 1976; Dyrynda, 1994). The abundance of bryozoans is positively correlated with supply of stable hard substrata and hence with current strength (Eggleston, 1972b; Ryland, 1976).This biotope and the species it supports are characteristic of moderate to strong currents, subject to different degrees of sediment scour and silt. The different sub-biotopes differ in the degree of siltation and scour to which they are exposed. For example, MCR.Flu.Flu, is species poor and exposed to high silt levels. Sponges, hydroids and ascidians increase in number with decreasing silt, the sub-biotopes differing in their degree of wave exposure or water flow rates. The distribution of the sub-biotopes is also dependant on their geographic position, e.g. MCR.Flu.Flu predominates on the eastern coast Scotland and England, while MCR.Flu.Hocu is only found in south England (Connor et al, 1997; Hartnoll, 1998).
Species composition
Species found especially in this biotope
- Abietinaria abietina
- Alcyonidium parasiticum
- Chartella papyracea
- Eucratea loricata
- Flustra foliacea
- Hyas coarctatus
- Jujubinus miliaris
- Nemertesia antennina
- Nemertesia ramosa
- Polymastia boletiformis
- Polymastia mamillaris
- Sertularia argentea
- Thuiaria thuja
- Tubularia indivisa
- Vesicularia spinosa
Rare or scarce species associated with this biotope
-
Additional information
The Flustra foliacea dominated biotopes support a large number of sessile, interstitial, and mobile cryptofauna. The species richness varies between sub-biotopes. For example the MNCR identified 183 species in MCR.Flu, 378 species in MCR.Flu.Flu, 664 in MCR.Flu.HByS, 305 in MCR.Flu.Hocu, and 594 in MCR.Flu.SerHyd (JNCC, 1999). It should be remembered that the above numbers are probably underestimates and that not all species occur in all instances of the biotope. However, the above estimates of species richness give an indication of the biodiversity of circalittoral faunal turf habitats.Sensitivity review
The MarLIN sensitivity assessment approach used below has been superseded by the MarESA (Marine Evidence-based Sensitivity Assessment) approach (see menu). The MarLIN approach was used for assessments from 1999-2010. The MarESA approach reflects the recent conservation imperatives and terminology and is used for sensitivity assessments from 2014 onwards.
Explanation
This biotope is dominated by the erect bryozoan Flustra foliacea, which if lost would result in loss of the biotope as described. The biotope is characterized by a faunal turf of hydroids and bryozoans. Although, loss of a single species may not be detrimental, loss of the bryozoan/ hydroid turf would result in degradation of the community, and potentially loss of the biotope as described. Therefore, Nemertesia ramosa has been included to represent Nemertesia species and other hydroids, while Bugulina turbinata has been included to represent Bugula species and other seasonal bryozoan species. The soft coral Alcyonium digitatum is included to represent the sensitivity of large epifauna and, together with Urticina felina, the sensitivity of anthozoans. Sebens (1985, 1986) demonstrated that predators, especially by sea urchins, were an important factor structuring epifaunal communities, therefore, Echinus esculentus has been included as important functional. The sensitivity of ascidians has been represented by Molgula manhattensis and the sensitivity of sponges by Halichondria panicea.Species indicative of sensitivity
Community Importance | Species name | Common Name |
Important other | Alcyonium digitatum | Dead man's fingers |
Important characterizing | Bugula turbinata | An erect bryozoan |
Important functional | Echinus esculentus | Edible sea urchin |
Important characterizing | Flustra foliacea | Hornwrack |
Important other | Halichondria panicea | Breadcrumb sponge |
Important other | Molgula manhattensis | A sea squirt |
Important characterizing | Nemertesia ramosa | A hydroid |
Important other | Urticina felina | Dahlia anemone |
Physical Pressures
Use [show more] / [show less] to open/close text displayed
Intolerance | Recoverability | Sensitivity | Species Richness | Evidence / Confidence | |
Substratum Loss [Show more]Removal of the substratum will result in removal of all the sessile attached species, together with most of the slow mobile species (crustaceans, sea urchins and starfish) and an intolerance of high has been recorded. Recoverability will depend on recruitment from neighbouring communities and subsequent recovery of the original abundance of species, which may take many years, especially in slow growing sponges, anthozoans and Flustra foliacea. Therefore, a recoverability of high has been recorded (see additional information below). | High | High | Moderate | Major decline | Moderate |
Smothering [Show more]This biotope is characteristic of areas subject to sediment scour and siltation. Holme & Wilson (1985) reported Flustra foliacea dominated communities that were subject to periodic smothering by thin layers of sand, up to ca 5cm in the central English Channel. Flustra foliacea and hydroids such as Nemertesiaspp. and Tubularia sp., the bryozoan Vesicularia spinosa, the ascidians Ascidia mentula and Dendrodoa grossularia and the anemone Urticina felina were noted in their sand scoured communities. Smothering with a layer of sediment will prevent or reduce feeding and hence growth and reproduction. Although the biotope will probably survive smothering at the benchmark level, the species richness of the biotope will probably decline due to the loss of more intolerant species such as the bryozoan Bugula spp., sponges (e.g. Halichondria panicea) some ascidians (e.g. Clavelina lepadiformis) and reduced abundance of Alcyonium digitatum and the ascidian Molgula manhattensis, due to clogging of their filtration apparatus, interrupted feeding and hence reduced growth, and potential short term anoxia under the sediment layer. Also, associated small species such as prosobranchs, amphipods and worms may be intolerant. Therefore, an intolerance of intermediate is suggested to reflect the reduced species richness. Recoverability is likely to be high (see additional information below). Prolonged smothering, however, is likely to favour biotopes dominated by Urticina felina (e.g. Centrophorus granulosus). | Intermediate | High | Low | Major decline | Low |
Increase in suspended sediment [Show more]This biotope is characteristic of areas subject to sediment scour and suspended sediment. In areas of high suspended sediment and siltation along the Northumberland coast, the MCR.Flu biotope is represented by a relatively species poor sub-biotope A4.2141, characterized by the presence of Thuiaria thuja and Sabellaria spinulosa. While an increase in suspended sediment at the benchmark level for a month is likely to reduce the efficiency of filter feeding in some species (e.g. bryozoans, hydroids and soft corals), most species are likely to survive for a month. If there is an associated increase in siltation, it is likely to interfere with larval growth and settlement if it coincided with the reproductive season. Therefore, an intolerance of low has been recorded. If siltation was prolonged then species richness may decrease, especially in more intolerant ascidians and bryozoans. | Low | Very high | Very Low | Minor decline | Low |
Decrease in suspended sediment [Show more]This biotope is characteristic of areas subject to sediment scour and suspended sediment. The relatively species poor sub-biotope A4.2141 is characteristic of high levels of suspended sediment. Therefore, with decreasing suspended sediment levels, species richness is likely to increase, to something like that of the other sub-biotopes (A4.137, MCR.Flu.HByS, and A5.444). A decrease in suspended sediment may decrease food availability for the duration of the benchmark (one month) but otherwise not adversely affect the biotope in such a short period of time. Therefore, an intolerance of low has been recorded. Prolonged decreases in suspended sediment, and consequent reduced scour may allow other species to colonize the habitat and out-compete characterizing species, perhaps increasing dominance by ascidians, sponges or anemones, and their biotopes. | Low | Moderate | Not relevant | Moderate | |
Dessication [Show more]Bryozoans, hydroids, sponges, and soft corals, are probably highly intolerance of desiccation. However, this biotope is circalittoral, occurring below 5-10m depth and possibly to great depths (e.g. ca 200m) (see Flustra foliacea review) and unlikely to be exposed to the air and desiccation. | Not relevant | Not relevant | Not relevant | Not relevant | High |
Increase in emergence regime [Show more]An increase or decrease in tidal emergence is unlikely to affect circalittoral habitats, except that the influence of wave action may be increased (see wave action below). | Not relevant | Not relevant | Not relevant | Not relevant | Not relevant |
Decrease in emergence regime [Show more]An increase or decrease in tidal emergence is unlikely to affect circalittoral habitats, except that the influence of wave action may be decreased (see wave action below). | Not sensitive* | Not relevant | |||
Increase in water flow rate [Show more]This biotope is characterized by species that are tolerant of moderately strong to strong tidal streams and associated sediment scour. Flustra foliacea colonies are flexible, robust and reach high abundances in areas subject to strong tidal streams (Stebbing, 1971a; Eggleston, 1972b; Knight-Jones & Nelson-Smith, 1977; Hiscock, 1983, 1985; Holme & Wilson, 1985) and occur in areas subject to very strong tidal streams. While Flustra foliacea may not be adversely affected by an increase in water flow to very strong, other species in the biotope such as hydroids and erect bryozoans may be adversely affected by the physical drag caused by very strong water flow, e.g. Bugula species or Molgula manhattensis. Increased water flow is likely to reduce predation by Asterias rubens and large sea urchins, e.g. Echinus esculentus was observed to be rolled along the substratum by currents of 2.6 knots or above (Comely & Ansell, 1988). But the increased sediment scour likely to accompany increased water flow rates may be more damaging, resulting in an increase in the extent of biotopes found in higher scour, such as found at the sediment /rock interface, e.g. Urticina felina dominated Centrophorus granulosus. In severe scour, the community may become impoverished, consisting of Pomatoceros spp., encrusting bryozoans, encrusting coralline algae and Balanus crenatus, e.g. A5.141. Where the biotopes occur on stones or boulders, increased water flow may result in movement or rolling of the stones and boulders, and hence severe scour and abrasion. The likely associated scour and displacement of some species in the biotope over the year (see benchmark), is likely to change the biotope to a different one. Therefore, an intolerance of high has been recorded. Recoverability is likely to be high (see additional information below). | High | High | Moderate | Decline | Low |
Decrease in water flow rate [Show more]This biotope is characterized by species that are tolerant of moderately strong to strong tidal streams and associated sediment scour. A decrease in water flow rates will decrease sediment scour, however, in the proximity of sediment is likely to result in greater siltation. Water movement is essential for suspension feeders such as hydroids, bryozoans, sponges, amphipods and ascidians to supply adequate food, remove metabolic waste products, prevent accumulation of sediment and disperse larvae or medusae. In addition, water flow was shown to be important for the supply of suitable hard substrata for colonization, and hence the development of bryozoan communities (Eggleston, 1972b; Ryland, 1976). Hydroids are also expected to be abundant where water movement is sufficient to supply adequate food but not cause damage (Hiscock, 1983; Gili & Hughes, 1995). For example, Sertularia operculata was observed to die within a few months when transplanted from Lough Ine rapids to sheltered water, due to the build up of a layer of silt (Round, et al., 1961). Therefore, a decrease in water flow from e.g. moderately strong to very weak is likely to encourage colonization by other species of hydroids, ascidians, sponges and anemones, and may increase the risk of sea urchin predation, resulting in significant changes in the community and possibly the loss of the dominant hydroid/ bryozoans turf. Therefore, an intolerance of high has been recorded. Recoverability is likely to take up to 5 years (see additional information below). | Low | Very high | Moderate | Minor decline | Low |
Increase in temperature [Show more]Growth rates were reported to increase with temperature in several bryozoan species, however, zooid size decreased, which may be due to increased metabolic costs at higher temperature (Menon, 1972; Ryland, 1976; Hunter & Hughes, 1994). Temperature is also a critical factor stimulating or inhibiting reproduction in hydroids, most of which have an optimum temperature range for reproduction (Gili & Hughes, 1995). Most of the hydroid and bryozoan species within the biotope are recorded to the north or south of the British Isles and are unlikely to be adversely affected by long term increases in temperature at the benchmark level. Similarly, sponges of the species Polymastia occurs from the Arctic to Gibraltar, and Haliclona oculata is widespread. However, the hydroid Thuiaria thuja is a primarily northern species and likely to be lost due to long term changes in temperature (Hiscock et al., 2001). Similarly, while not likely to be adversely affected by long term change, Urticina felina and Echinus esculentus are probably intolerance of short term increases in temperature at the benchmark level. However, circalittoral habitats are probably protected from extreme changes in temperature by their depth. Whilst the northern hydroid Thuiaria thuja may be lost from the biotope, southern species may take its place and, in view of the likely favourable effects on growth rates in other species, an overall rank of not sensitive has been recorded. | Tolerant | Not relevant | Not relevant | Minor decline | Low |
Decrease in temperature [Show more]The majority of the dominant or characterizing species in the biotope are boreal or have a wide distribution to the north or south of British and Ireland and the biotope is unlikely to be adversely affected by long term changes in temperature at the benchmark level. Short term acute change may adversely affect some species, e.g. Echinus esculentus and Urticina felina, resulting in reduced extent or abundance. In addition, temperature influences growth and reproduction in many species of hydroids, bryozoans and ascidians (see above and species reviews). Therefore, an intolerance of low has been recorded. | Low | Very high | Moderate | Minor decline | Low |
Increase in turbidity [Show more]An increase in turbidity is likely to result in a decrease in phytoplankton and macroalgal primary production, which may reduce food available to the suspension feeders within the community. As a result , growth rates and reproduction may be decreased, and some species may not be able to keep up with predation (e.g. see Gaulin et al., 1986). However, slow growing species such as Flustra foliacea can probably survive reductions in food availability for a year. Therefore, an intolerance of low has been recorded. | Low | Very high | Very Low | Minor decline | Low |
Decrease in turbidity [Show more]A decrease in turbidity may increase phytoplankton and hence zooplankton productivity and potentially increase food availability. Increased light penetration may allow macroalgae to colonize deeper water. Macroalgae effectively compete for space and grow over and may smother fauna. Therefore, decreased turbidity may allow macroalgae to colonize the more shallow examples of this biotope, resulting in loss of a proportion of the biotope, although some members of the community are likely to survive even in the presence of macroalgae. The favourable effects of a potential increase in food supply are probably more important than overgrowth by macroalgae at shallow depths. Therefore a rank of not sensitive has been recorded. | Intermediate | High | Low | Decline | Low |
Increase in wave exposure [Show more]This biotope occurs in moderately wave exposed habitats. The sub-biotope MCR.Flu.HByS is also found in wave exposed habitats and includes robust hydroids (e.g. Nemertesia antennina, and Abietinaria abietina) and sponges such as Dysidea fragilis, Polymastia boletiformis and Cliona celata (Conner et al., 1997a). The oscillatory flow generated by wave action is potentially more damaging than unidirectional flow but is attenuated with depth (Hiscock, 1983). Many of the species in the biotope are likely to be able to tolerate an increase in wave exposure from moderately exposed to very exposed, for example, Alcyonium digitatum, Urticina felina, Bugula species, the sponges Halichondria panicea and Esperiopsis fucorum, and probably the hydroids Nemertesia antennina and Sertularia argentea Abietinaria abietina. Flustra foliacea is found in very wave exposed site, although probably in deeper waters. However, less flexible or weaker hydroids and bryozoans may be removed, e.g. Nemertesia ramosa. Increased wave action may decrease sea urchin and starfish predation, perhaps allowing larger, massive species (e.g. sponges, anemones and ascidians) increase in dominance. Therefore, it is likely that some species within the biotope, especially hydroids may be lost, and some of the Flustra foliacea turf may also be damaged and an intolerance of intermediate has been recorded. Recoverability is likely to be high (see additional information below). | Intermediate | High | Low | Minor decline | Low |
Decrease in wave exposure [Show more]The strong tidal streams that typify this biotope are probably more important as water movement than wave induced oscillatory flow. Therefore, a decrease in wave action may allow more delicate species, such as Nemertesia ramosa, ascidians and sponges to increase in abundance. Decreased wave action may allow the biotope to extend into shallower water (e.g. A4.137). But reduced wave action may result in an increase in sea urchin predation and hence increased patchiness and species richness (Sebens, 1985; Hartnoll, 1998). Overall, a decrease in wave action may not adversely affect the biotope while strong tidal flow maintains adequate water exchange and, although some species in the biotope may change, Flustra foliacea and the biotope will probably survive. Therefore, an intolerance of low has been recorded. | Low | Very high | Moderate | Minor decline | Low |
Noise [Show more]Hydroids, bryozoans, sponges and ascidians are unlikely to be sensitive to noise or vibration at the benchmark level. Mobile fish species may be temporarily scared away from the areas but few if any adverse effects on the biotope are likely to result. | Tolerant | Not relevant | Not relevant | Not relevant | High |
Visual Presence [Show more]Hydroid and bryozoan polyps or barnacle cirri may retract when shaded by potential predators, however the community is unlikely to be affected by visual presence. Mobile fish species may be temporarily scared away from the areas but few if any adverse effects on the biotope are likely to result. | Tolerant | Not relevant | Not relevant | Not relevant | High |
Abrasion & physical disturbance [Show more]The species that characterize this biotope are tolerant of sediment scour and unlikely to be damaged by abrasion. However, physical disturbance by an anchor or mobile fishing gear may be more damaging. Erect epifaunal species are particularly vulnerable to physical disturbance. Hydroids and bryozoans are likely to be detached or damaged by bottom trawling or dredging (Holt et al., 1995). Veale et al. (2000) reported that the abundance, biomass and production of epifaunal assemblages decreased with increasing fishing effort. Hydroid and bryozoan matrices were reported to be greatly reduced in fished areas (Jennings & Kaiser, 1998 and references therein). Mobile gears also result in modification of the substratum, including removal of shell debris, cobbles and rocks, and the movement of boulders (Bullimore, 1985; Jennings & Kaiser, 1998). The removal of rocks or boulders to which species are attached results in substratum loss (see above). Magorrian & Service (1998) reported that queen scallop trawling flattened horse mussel beds and removed emergent epifauna in Strangford Lough. They suggested that the emergent epifauna such as Alcyonium digitatum, a frequent component of this biotope, were more intolerant than the horse mussels themselves and reflected early signs of damage. However, Alcyonium digitatum is more abundant on high fishing effort grounds, which suggests that this seemingly fragile species is more resistant to abrasive disturbance than might be assumed (Bradshaw et al., 2000), presumably owing to good recovery due to its ability to replace senescent cells and regenerate damaged tissue, together with early larval colonization of available substrata. Species with fragile tests such as Echinus esculentus and the brittlestar Ophiocomina nigra and edible crabs Cancer pagurus were reported to suffer badly from the impact of a passing scallop dredge (Bradshaw et al., 2000). Scavengers such as Asterias rubens and Buccinum undatum were reported to be fairly robust to encounters with trawls (Kaiser & Spencer, 1995) may benefit in the short term, feeding on species damaged or killed by passing dredges. However, Veale et al. (2000) did not detect any net benefit at the population level. Overall, physical disturbance by an anchor or mobile fishing gear is likely to remove a proportion of all groups within the community and attract scavengers to the community in the short term. Therefore, an intolerance of intermediate has been recorded. Recoverability is likely to be high due to repair and regrowth of hydroids and bryozoans (e.g. Flustra foliacea), and recruitment within the community from surviving colonies and individuals (see additional information below). | Intermediate | High | Low | Decline | Moderate |
Displacement [Show more]Most permanently fixed, sessile species, such as bryozoans (e.g. Flustra foliacea and Bugula species), sponges (e.g. Halichondria panicea), ascidians (e.g. Molgula manhattensis) and hydroids (e.g. Nemertesia species) cannot reattach to the substratum if removed, and may be damaged or destroyed in the process. Hydroids and sponges may be able to grow from fragments, aiding recovery. Mobile species, such as amphipods, gastropods, small crustaceans, crabs and fish are likely to survive displacement. Anemones (e.g. Urticina felina) are strongly but not permanently attached and will probably reattach to suitable substrata. However, the dominant bryozoans and hydroids are likely to be lost and an intolerance of high has been recorded. Recovery of the Flustra foliacea abundance is likely to take many years and a recoverability of high has been recorded (see additional information below). | High | High | Moderate | Major decline | Moderate |
Chemical Pressures
Use [show more] / [show less] to open/close text displayed
Intolerance | Recoverability | Sensitivity | Richness | Evidence / Confidence | |
Synthetic compound contamination [Show more]Bryozoans are common members of the fouling community, and amongst those organisms most resistant to antifouling measures, such as copper containing anti-fouling paints (Soule & Soule, 1979; Holt et al., 1995). However, Hoare & Hiscock (1974) suggested that Polyzoa (Bryozoa) were amongst the most intolerant species to acidified halogenated effluents in Amlwch Bay, Anglesey and reported that Flustra foliacea did not occur less than 165m from the effluent source and noted that Bugulina flabellata did not occur within the bay. Urticina felina survived near to the acidified halogenated effluent discharge in a 'transition' zone where many other species were unable to survive, suggesting a tolerance to chemical contamination but did not survive closer to the effluent source (Hoare & Hiscock, 1974). Moran & Grant (1993) reported that settlement of marine fouling species, including Bugula neritina was significantly reduced in Port Kembla Harbour, Australia, exposed to high levels of cyanide, ammonia and phenolics. The species richness of hydroid communities decreases with increasing pollution (Boero, 1984; Gili & Hughes, 1995). Stebbing (1981) reported that Cu, Cd, and tributyl tin fluoride affected growth regulators in Laomedea (as Campanularia) flexuosa resulting in increased growth. Alcyonium digitatum at a depth of 16m in the locality of Sennen Cove (Pedu-men-du, Cornwall) died resulting from the offshore spread and toxic effect of detergents e.g. BP 1002 sprayed along the shoreline to disperse oil from the Torrey Canyon tanker spill (Smith, 1986). Possible sub-lethal effects of exposure to synthetic chemicals, may result in a change in morphology, growth rate or disruption of reproductive cycle. Smith (1968) also noted that large numbers of dead Echinus esculentus were found between 5.5 and 14.5 m in the vicinity of Sennen, presumably due to a combination of wave exposure and heavy spraying of dispersants in that area (Smith, 1968). Smith (1968) also demonstrated that 0.5 -1ppm of the detergent BP1002 resulted in developmental abnormalities in echinopluteus larvae of Echinus esculentus. Tri-butyl tin (TBT) has a marked effect on numerous marine organisms (Bryan & Gibbs, 1991). The encrusting bryozoan Schizoporella errata suffered 50% mortality when exposed for 63 days to 100ng/l TBT. Bryan & Gibbs (1991) reported that virtually no hydroids were present on hard bottom communities in TBT contaminated sites and suggested that some hydroids were intolerant of TBT levels between 100 and 500 ng/l. Copepod and mysid crustaceans were particularly intolerant of TBT while crabs were more resistant (Bryan & Gibbs, 1991), although recent evidence suggests some sublethal endocrine disruption in crabs. The effect of TBT on Nucella lapillus and other neogastropods is well known (see review), and similar effects on reproduction may occur in other gastropod molluscs, including nudibranchs. Rees et al. (2001) reported that the abundance of epifauna had increased in the Crouch estuary in the five years since TBT was banned from use on small vessels. Rees et al. (2001) suggested that TBT inhibited settlement in ascidian larvae. This report suggests that epifaunal species (including, bryozoan, hydroids and ascidians) may be at least inhibited by the presence of TBT. Therefore, hydroids crustaceans, gastropods, and ascidians are probably intolerant of TBT contamination while bryozoans are probably intolerant of other chemical pollution and an intolerance of intermediate has been recorded, albeit at low confidence. A recoverability of moderate has been recorded (see additional information below). | Intermediate | High | Low | Decline | Low |
Heavy metal contamination [Show more]Various heavy metals have been show to have sublethal effects on growth in the few hydroids studied experimentally (Stebbing, 1981; Bryan, 1984; Ringelband, 2001). Bryozoans are common members of the fouling community and amongst those organisms most resistant to anti-fouling measures, such as copper containing anti-fouling paints. Bryozoans were also shown to bioaccumulate heavy metals to a certain extent (Soule & Soule, 1979; Holt et al., 1995). However, Bugula neritina was reported to survive but not grow exposed to ionic Cu concentrations of 0.2-0.3 ppm (larvae died above 0.3ppm) but die where the surface leaching rate of Cu exceeded 10µg Cu/cm²/day (Ryland, 1967; Soule & Soule, 1979). Ryland (1967) also noted that Bugula neritina was less intolerant of Hg than Cu. Echinus esculentus populations in the vicinity of an oil terminal in A Coruna Bay, Spain, showed developmental abnormalities in the skeleton and their tissues contained high levels of aliphatic hydrocarbons, naphthalenes, pesticides and heavy metals (Zn, Hg, Cd, Pb, and Cu) (Gomez & Miguez-Rodriguez 1999). Waters containing 25 µg / l Cu caused developmental disturbances in Echinus esculentus (Kinne, 1984) and heavy metals caused reproductive anomalies in the starfish Asterias rubens (Besten, et al., 1989, 1991). Sea urchin larvae have been used in toxicity testing and as a sensitive assay for water quality (reviewed by Dinnel et al. 1988), so that echinoderms are probably intolerant of a heavy metal contamination. Gastropod molluscs have been reported to relatively tolerant of heavy metals while a wide range of sublethal and lethal effects have been observed in larval and adult crustaceans (Bryan, 1984). Overall, the dominant bryozoans may be tolerant and hydroids manifest only sublethal effects. The sea urchin Echinus esculentus is probably highly intolerant of heavy metal contamination. Heavy metals contamination may, therefore, reduce reproduction and recruitment in starfish and sea urchins, potentially reducing predation pressure in the biotope. Therefore, an intolerance of low has been recorded to represent the sublethal effects on dominant bryozoans and hydroids. Loss of predatory sea urchins, may result in an increased dominance by some species and a slight decrease in species richness. | Low | Very high | Very Low | Minor decline | Very low |
Hydrocarbon contamination [Show more]Flustra foliacea dominated communities are likely to be protected from the direct effects of oil spills by its subtidal habit but may be exposed to emulsified oil treated with dispersants, especially in areas of turbulence, or exposed to water soluble fractions of oils, PAHs or oil adsorbed onto particulates. For example:
If the physiology within different animals groups can be assumed to be similar, then bryozoans, amphipods, echinoderms and soft corals may be intolerant of hydrocarbon contamination, while hydroids may demonstrate sublethal effects and anemones and some species of sponge are relatively tolerant. Some members of the bryozoan turf and some members of the community may be lost or damaged as a result of acute hydrocarbon contamination, although a recognisable biotope may remain. Therefore, an intolerance of intermediate has been suggested, albeit at very low confidence. Recoverability is likely to be moderate (see additional information below). | Intermediate | High | Low | Decline | Very low |
Radionuclide contamination [Show more]No information found. | No information | Not relevant | No information | Insufficient information |
Not relevant |
Changes in nutrient levels [Show more]An increase in nutrient levels from e.g. sewage sludge, sewage effluent or riverine flooding, may result in an increase in inorganic and organic suspended particulates (see above), increased turbidity (see above) and increased phytoplankton productivity. Moderate nutrient enrichment may increase the food available to the community in the form of phytoplankton, zooplankton or organic particulates. However, eutrophication may result in deoxygenation (see below) or algal blooms. While the biotope is unlikely to be directly affected by algal blooms, the biotope may be adversely affected by toxins from toxic algae that accumulate in zooplankton, or smothered by dead 'bloom' algae and deoxygenation resulting form their subsequent decay (see below). Death of a bloom of the phytoplankton Gyrodinium aureolum in Mounts Bay, Penzance in 1978 produced a layer of brown slime on the sea bottom. This resulted in the death of invertebrates, including Echinus esculentus, Marthasterias glacialis, while sessile bryozoans, sponges and Alcyonium spp. appeared moribund, presumably due to anoxia caused by the decay of the dead dinoflagellates (Griffiths et al. 1979). This biotope occurs in areas subject to moderately strong to strong tidal streams, so that prolonged deoxygenation is unlikely to occur. However, an intolerance of low has been recorded to represent the potential toxic effects of the algae and the siltation caused by death of an algal bloom. | Low | Very high | Very Low | No change | Low |
Increase in salinity [Show more]This biotope occurs in full salinity and is unlikely to encounter increases in salinity. | Not relevant | Not relevant | Not relevant | Not relevant | High |
Decrease in salinity [Show more]Most of the species identified as indicative of intolerance may be of 'intermediate' or 'low' intolerance to a reduction in salinity. Ryland (1970) stated that, with a few exceptions, the Gymnolaemata were fairly stenohaline and restricted to full salinity (ca 35 psu) and noted that reduced salinities result in an impoverished bryozoan fauna but this biotope (MCR.Flu) and those biotopes it has been used to represent, are found in the circalittoral and are unlikely to be exposed to reduced or low salinity. | Not sensitive* | Not relevant | Moderate | ||
Changes in oxygenation [Show more]This biotope occurs in areas subject to moderately strong to strong tidal streams, so that deoxygenating conditions are unlikely to develop. | Not relevant | Not relevant | Not relevant | Not relevant | Moderate |
Biological Pressures
Use [show more] / [show less] to open/close text displayed
Intolerance | Recoverability | Sensitivity | Richness | Evidence / Confidence | |
Introduction of microbial pathogens/parasites [Show more]Stebbing (1971a) reported that encrusting epizoics reduced the growth rate of Flustra foliacea by ca 50% and Stebbing (1971b) described the epizoic fauna of horn wrack in detail. For example, Bugulina flabellata produces stolons that grow in and through the zooids of Flustra foliacea, causing "irreversible degeneration of the enclosed polypide" (Stebbing, 1971b). Therefore, given the reduction in growth caused by epizoic infestation an intolerance of low has been recorded. Recovery and repair would probably be rapid (see additional information below). | Low | Very high | Very Low | No change | Moderate |
Introduction of non-native species [Show more]No non-native species are known to occur in this biotope. | No information | Not relevant | No information | Insufficient information |
Not relevant |
Extraction of this species [Show more]Flustra foliacea is not presently known to be subject to extraction. However, many bryozoans have been recently found to contain pharmacologically active substances (Hayward & Ryland, 1998) and, therefore, Flustra foliacea, Bugula spp. and other bryozoans may be subject to harvesting in the future. The use of mobile fishing gear, such as scallop dredges and beam trawls, in the vicinity of the biotope result in physical disturbance to the sediment surface, and an increase in suspended sediment (Hartnoll, 1998). Circalittoral faunal turf biotopes may be subject to fishing for crabs, crawfish and lobster, using pots, creels or fixed bottom-set tangle or gill nets (Hartnoll, 1998). Potting and fixed netting (their placement and collection) probably results in abrasion and physical disturbance (see above). In addition, Echinus esculentus has been collected by diving in the past (Nichols, 1984). Loss of functionally important predators such as sea urchins, and to a lesser extent crabs and lobster may affect community structure (Hartnoll, 1998). Therefore, an intolerance of intermediate and a recoverably of high have been recorded to represent physical disturbance caused by fishing activities (see additional information below). | Intermediate | High | Low | Minor decline | Low |
Extraction of other species [Show more] | Intermediate | High | Low | Minor decline | Low |
Additional information
Recoverability
Where local populations exist or remain after disturbance recruitment is likely to be rapid for most species, including Flustra foliacea. Many species, e.g. hydroids, colonial ascidians, sponges and Metridium senile are capable of asexual reproduction and colonize space rapidly. For example, in studies of subtidal epifaunal communities in New England, Sebens (1985, 1986) reported that cleared areas were colonized by erect hydroids, bryozoans, crustose red algae and tube worms within 1-4 months in spring, summer and autumn. Tunicates such as Dendrodoa carnea and Aplidium spp. appeared within a year, Aplidium sp., and Halichondria panicea achieved pre-clearance cover within >2 years, while only a few individuals of Metridium senile and Alcyonium sp. colonized within 4 years.
Flustra foliacea is slow growing, long-lived and new colonies take at least 1 year to develop erect growth and 1-2 years to reach maturity (Stebbing, 1971a; Eggleston, 1972a), depending on environmental conditions. Four years after sinking, the wreck of a small coaster, the M.V. Robert, off Lundy was found to be colonized by erect bryozoans and hydroids, including occasional Flustra foliacea (Hiscock, 1981). The wreck was several hundreds of metres from any significant hard substrata, and hence a considerable distance from potentially parent colonies (Hiscock, 1981 and pers. comm.). Overall, local recruitment is probably good and a damaged or reduced population of Flustra foliacea, other erect bryozoans and hydroids may recover abundance and percentage cover in less than 5 years.
Where the populations are removed or destroyed. recolonization will depend on recruitment of larvae from other communities. The majority of species are widespread but have poor dispersal so that recruitment rates will depend on the proximity of nearby communities and the hydrographic regime. Exceptions include, mobile crustaceans and echinoderms with long-lived planktonic larvae, and Nemertesia antennina and Alcyonium digitatum which can probably disperse up to 50 m or over 100 km respectively (Hughes, 1977; Hartnoll, 1998). But Sebens (1985) suggested that Alcyonium spp. and Metridium senile would probably not recruit to epifaunal communities unless other populations of the species were nearby. Flustra foliacea is evidently capable of dispersing over considerable distance, since it colonized the M.V. Robert and achieved 1-5% (occasional) cover within 4 years (Hiscock, 1981). However, it would probably take many years for Flustra foliacea to recover its original cover. Many other members of the community would probably occupy space rapidly once they colonize the habitat.
Colonization of cleared space from distant populations is probably stochastic, reliant on hydrography and environmental conditions. Overall, encrusting bryozoans, hydroids, and ascidians will probably develop a faunal turf within less than 2 years, and Flustra foliacea can evidently colonize and reach an abundance of occasional (1-5% cover) within 4 years. While the biotope may be recognisable in up to five years, Flustra foliacea may take at least five years to recover its original dominance. Where habitats are isolated by geography (distance) or hydrography, recovery may take longer.
Bibliography
Besten, P.J. den, Donselaar, E.G. van, Herwig, H.J., Zandee, D.I. & Voogt, P.A., 1991. Effects of cadmium on gametogenesis in the seastar Asterias rubens L. Aquatic Toxicology, 20, 83-94.
Besten, P.J. den, Herwig, H.J., Zandee, D.I. & Voogt, P.A., 1989. Effects of Cd and PCBs on reproduction in the starfish Asterias rubens: aberrations in early development. Ecotoxicology and Environmental Safety, 18, 173-180.
Bishop, G.M. & Earll, R., 1984. Studies on the populations of Echinus esculentus at the St Abbs and Skomer voluntary Marine Nature Reserves. Progress in Underwater Science, 9, 53-66.
Boero, F., 1984. The ecology of marine hydroids and effects of environmental factors: a review. Marine Ecology, 5, 93-118.
Bradshaw, C., Veale, L.O., Hill, A.S. & Brand, A.R., 2000. The effects of scallop dredging on gravelly seabed communities. In: Effects of fishing on non-target species and habitats (ed. M.J. Kaiser & de S.J. Groot), pp. 83-104. Oxford: Blackwell Science.
Brault, S. & Bourget, E., 1985. Structural changes in an estuarine subtidal epibenthic community: biotic and physical causes. Marine Ecology Progress Series, 21, 63-73.
Brazier, P., Davies, J., Holt, R. & Murray, E., 1998. Marine Nature Conservation Review Sector 5. South-east Scotland and north-east England: area summaries. Peterborough: Joint Nature Conservation Committee. [Coasts and Seas of the United Kingdom. MNCR Series]
Bryan, G.W. & Gibbs, P.E., 1991. Impact of low concentrations of tributyltin (TBT) on marine organisms: a review. In: Metal ecotoxicology: concepts and applications (ed. M.C. Newman & A.W. McIntosh), pp. 323-361. Boston: Lewis Publishers Inc.
Bryan, G.W., 1984. Pollution due to heavy metals and their compounds. In Marine Ecology: A Comprehensive, Integrated Treatise on Life in the Oceans and Coastal Waters, vol. 5. Ocean Management, part 3, (ed. O. Kinne), pp.1289-1431. New York: John Wiley & Sons.
Bullimore, B., 1985. An investigation into the effects of scallop dredging within the Skomer Marine Reserve. Report to the Nature Conservancy Council by the Skomer Marine Reserve Subtidal Monitoring Project, S.M.R.S.M.P. Report, no 3., Nature Conservancy Council.
Castric-Fey, A., 1974. Les peuplements sessiles du benthos rocheux de l'archipel de Glenan (Sud-Bretagne). Ecologie descriptive and experimentale. , Ph. D. thesis, Université de Bretagne Occidentale, L' Université Paris, Paris, France.
Comely, C.A. & Ansell, A.D., 1988. Invertebrate associates of the sea urchin, Echinus esculentus L., from the Scottish west coast. Ophelia, 28, 111-137.
Connor, D.W., Brazier, D.P., Hill, T.O., & Northen, K.O., 1997b. Marine biotope classification for Britain and Ireland. Vol. 1. Littoral biotopes. Joint Nature Conservation Committee, Peterborough, JNCC Report no. 229, Version 97.06., Joint Nature Conservation Committee, Peterborough, JNCC Report No. 230, Version 97.06.
Cornelius, P.F.S., 1992. Medusa loss in leptolid Hydrozoa (Cnidaria), hydroid rafting, and abbreviated life-cycles among their remote island faunae: an interim review.
Davies, C.E. & Moss, D., 1998. European Union Nature Information System (EUNIS) Habitat Classification. Report to European Topic Centre on Nature Conservation from the Institute of Terrestrial Ecology, Monks Wood, Cambridgeshire. [Final draft with further revisions to marine habitats.], Brussels: European Environment Agency.
Dinnel, P.A., Pagano, G.G., & Oshido, P.S., 1988. A sea urchin test system for marine environmental monitoring. In Echinoderm Biology. Proceedings of the Sixth International Echinoderm Conference, Victoria, 23-28 August 1987, (R.D. Burke, P.V. Mladenov, P. Lambert, Parsley, R.L. ed.), pp 611-619. Rotterdam: A.A. Balkema.
Dyrynda, P.E.J. & Ryland, J.S., 1982. Reproductive strategies and life histories in the cheilostome marine bryozoans Chartella papyracea and Bugula flabellata. Marine Biology, 71, 241-256.
Dyrynda, P.E.J., 1994. Hydrodynamic gradients and bryozoan distributions within an estuarine basin (Poole Harbour, UK). In Proceedings of the 9th International Bryozoology conference, Swansea, 1992. Biology and Palaeobiology of Bryozoans (ed. P.J. Hayward, J.S. Ryland & P.D. Taylor), pp.57-63. Fredensborg: Olsen & Olsen.
Eggleston, D., 1972a. Patterns of reproduction in marine Ectoprocta off the Isle of Man. Journal of Natural History, 6, 31-38.
Eggleston, D., 1972b. Factors influencing the distribution of sub-littoral ectoprocts off the south of the Isle of Man (Irish Sea). Journal of Natural History, 6, 247-260.
Gaulin, G., Dill, L., Beaulieu, J. & Harris, L.G., 1986. Predation-induced changes in growth form in a nudibranch-hydroid association. Veliger, 28, 389-393.
Gili, J-M. & Hughes, R.G., 1995. The ecology of marine benthic hydroids. Oceanography and Marine Biology: an Annual Review, 33, 351-426.
Gommez, J.L.C. & Miguez-Rodriguez, L.J., 1999. Effects of oil pollution on skeleton and tissues of Echinus esculentus L. 1758 (Echinodermata, Echinoidea) in a population of A Coruna Bay, Galicia, Spain. In Echinoderm Research 1998. Proceedings of the Fifth European Conference on Echinoderms, Milan, 7-12 September 1998, (ed. M.D.C. Carnevali & F. Bonasoro) pp. 439-447. Rotterdam: A.A. Balkema.
Gordon, D.P., 1972. Biological relationships of an intertidal bryozoan population. Journal of Natural History, 6, 503-514.
Griffiths, A.B., Dennis, R. & Potts, G.W., 1979. Mortality associated with a phytoplankton bloom off Penzance in Mounts Bay. Journal of the Marine Biological Association of the United Kingdom, 59, 515-528.
Hartnoll, R.G., 1983. Substratum. In Sublittoral ecology. The ecology of the shallow sublittoral benthos (ed. R. Earll & D.G. Erwin), pp. 97-124. Oxford: Clarendon Press.
Hartnoll, R.G., 1998. Circalittoral faunal turf biotopes: an overview of dynamics and sensitivity characteristics for conservation management of marine SACs, Volume VIII. Scottish Association of Marine Sciences, Oban, Scotland, 109 pp. [UK Marine SAC Project. Natura 2000 reports.] Available from: http://ukmpa.marinebiodiversity.org/uk_sacs/pdfs/circfaun.pdf
Hatcher, A.M., 1998. Epibenthic colonization patterns on slabs of stabilised coal-waste in Poole Bay, UK. Hydrobiologia, 367, 153-162.
Hayward, P.J. & Ryland, J.S. 1998. Cheilostomatous Bryozoa. Part 1. Aeteoidea - Cribrilinoidea. Shrewsbury: Field Studies Council. [Synopses of the British Fauna, no. 10. (2nd edition)]
Hiscock, K., 1981. Marine life on the wreck of the M.V. "Robert". Report of the Lundy Field Society, 32, 40-44.
Hiscock, K., 1983. Water movement. In Sublittoral ecology. The ecology of shallow sublittoral benthos (ed. R. Earll & D.G. Erwin), pp. 58-96. Oxford: Clarendon Press.
Hiscock, K., Southward, A., Tittley, I., Jory, A. & Hawkins, S., 2001. The impact of climate change on subtidal and intertidal benthic species in Scotland. Scottish National Heritage Research, Survey and Monitoring Report , no. 182., Edinburgh: Scottish National Heritage
Hoare, R. & Hiscock, K., 1974. An ecological survey of the rocky coast adjacent to the effluent of a bromine extraction plant. Estuarine and Coastal Marine Science, 2 (4), 329-348.
Holme, N.A. & Wilson, J.B., 1985. Faunas associated with longitudinal furrows and sand ribbons in a tide-swept area in the English Channel. Journal of the Marine Biological Association of the United Kingdom, 65, 1051-1072.
Holt, T.J., Jones, D.R., Hawkins, S.J. & Hartnoll, R.G., 1995. The sensitivity of marine communities to man induced change - a scoping report. Countryside Council for Wales, Bangor, Contract Science Report, no. 65.
Houghton, J.P., Lees, D.C., Driskell, W.B., Lindstrom & Mearns, A.J., 1996. Recovery of Prince William Sound intertidal epibiota from Exxon Valdez oiling and shoreline treatments, 1989 through 1992. In Proceedings of the Exxon Valdez Oil Spill Symposium. American Fisheries Society Symposium, no. 18, Anchorage, Alaska, USA, 2-5 February 1993, (ed. S.D. Rice, R.B. Spies, D.A., Wolfe & B.A. Wright), pp.379-411.
Hughes, R.G., 1977. Aspects of the biology and life-history of Nemertesia antennina (L.) (Hydrozoa: Plumulariidae). Journal of the Marine Biological Association of the United Kingdom, 57, 641-657.
Hunter, E. & Hughes, R.N., 1994. Influence of temperature, food ration and genotype on zooid size in Celleporella hyalina (L.). In Proceedings of the 9th International Bryozoology Conference, Swansea, 1992. Biology and Palaeobiology of Bryozoans (ed. P.J. Hayward, J.S. Ryland & P.D. Taylor), pp. 83-86. Fredensborg: Olsen & Olsen.
Jennings, S. & Kaiser, M.J., 1998. The effects of fishing on marine ecosystems. Advances in Marine Biology, 34, 201-352.
Jensen, A.C., Collins, K.J., Lockwood, A.P.M., Mallinson, J.J. & Turnpenny, W.H., 1994. Colonization and fishery potential of a coal-ash artificial reef, Poole Bay, United Kingdom. Bulletin of Marine Science, 55, 1263-1276.
JNCC (Joint Nature Conservation Committee), 2022. The Marine Habitat Classification for Britain and Ireland Version 22.04. [Date accessed]. Available from: https://mhc.jncc.gov.uk/
JNCC (Joint Nature Conservation Committee), 1999. Marine Environment Resource Mapping And Information Database (MERMAID): Marine Nature Conservation Review Survey Database. [on-line] http://www.jncc.gov.uk/mermaid
Kaiser, M.J. & Spencer, B.E., 1995. Survival of by-catch from a beam trawl. Marine Ecology Progress Series, 126, 31-38.
Knight-Jones, E.W. & Nelson-Smith, A., 1977. Sublittoral transects in the Menai Straits and Milford Haven. In Biology of benthic organisms (ed. B.F. Keegan, P. O Ceidigh & P.J.S. Broaden), pp. 379-390. Oxford: Pergamon Press.
Magorrian, B.H. & Service, M., 1998. Analysis of underwater visual data to identify the impact of physical disturbance on horse mussel (Modiolus modiolus) beds. Marine Pollution Bulletin, 36 (5), 354-359. DOI https://doi.org/10.1016/s0025-326x(97)00192-6
Menon, N.R., 1972. Heat tolerance, growth and regeneration in three North Sea bryozoans exposed to different constant temperatures. Marine Biology, 15, 1-11.
Michel, W.C. & Case, J.F., 1984. Effects of a water-soluble petroleum fraction on the behaviour of the hydroid coelenterate Tubularia crocea. Marine Environmental Research, 13, 161-176.
Michel, W.C., Sanfilippo, K. & Case, J.F., 1986. Drilling mud evoked hydranth shedding in the hydroid Tubularia crocea. Marine Pollution Bulletin, 17, 415-419.
Mohammad, M-B.M., 1974. Effect of chronic oil pollution on a polychaete. Marine Pollution Bulletin, 5, 21-24.
Moran, P.J. & Grant, T.R., 1993. Larval settlement of marine fouling organisms in polluted water from Port Kembla Harbour, Australia. Marine Pollution Bulletin, 26, 512-514.
Nichols, D., 1984. An investigation of the population dynamics of the common edible sea urchin (Echinus esculentus L.) in relation to species conservation management. Report to Department of the Environment and Nature Conservancy Council from the Department of Biological Sciences, University of Exeter.
Osman, R.W., 1977. The establishment and development of a marine epifaunal community. Ecological Monographs, 47, 37-63.
Rees, H.L., Waldock, R., Matthiessen, P. & Pendle, M.A., 2001. Improvements in the epifauna of the Crouch estuary (United Kingdom) following a decline in TBT concentrations. Marine Pollution Bulletin, 42, 137-144. DOI https://doi.org/10.1016/S0025-326X(00)00119-3
Ringelband, U., 2001. Salinity dependence of vanadium toxicity against the brackish water hydroid Cordylophora caspia. Ecotoxicology and Environmental Safety, 48, 18-26.
Round, F.E., Sloane, J.F., Ebling, F.J. & Kitching, J.A., 1961. The ecology of Lough Ine. X. The hydroid Sertularia operculata (L.) and its associated flora and fauna: effects of transference to sheltered water. Journal of Ecology, 49, 617-629.
Ryland, J.S., 1967. Polyzoa. Oceanography and Marine Biology: an Annual Review, 5, 343-369.
Ryland, J.S., 1970. Bryozoans. London: Hutchinson University Library.
Ryland, J.S., 1976. Physiology and ecology of marine bryozoans. Advances in Marine Biology, 14, 285-443.
Salvini-Plawen, L.V., 1972. Cnidaria as food sources for marine invertebrates. Cahiers de Biologie Marine, 13, 385-400.
Sandrock, S., Scharf, E-M., von Oertzen, J.A., 1991. Short-term changes in settlement of micro- and macro-fouling organisms in brackish waters. Acta Ichthyologica et Piscatoria, 21(Suppl.), 221-235.
Sebens, K.P., 1985. Community ecology of vertical rock walls in the Gulf of Maine: small-scale processes and alternative community states. In The Ecology of Rocky Coasts: essays presented to J.R. Lewis, D.Sc. (ed. P.G. Moore & R. Seed), pp. 346-371. London: Hodder & Stoughton Ltd.
Sebens, K.P., 1986. Spatial relationships among encrusting marine organisms in the New England subtidal zone. Ecological Monographs, 56, 73-96. DOI https://doi.org/10.2307/2937271
Smaal, A.C., 1994. Theme V: The response of benthic suspension feeders to environmental changes. The Oosterschelde Estuary (The Netherlands): A case study of a changing ecosystem. Hydrobiologia, 282-283, 355-357.
Smith, J.E. (ed.), 1968. 'Torrey Canyon'. Pollution and marine life. Cambridge: Cambridge University Press.
Sommer, C., 1992. Larval biology and dispersal of Eudendrium racemosum (Hydrozoa, Eudendriidae). Scientia Marina, 56, 205-211. [Proceedings of 2nd International Workshop of the Hydrozoan Society, Spain, September 1991. Aspects of hydrozoan biology (ed. J. Bouillon, F. Cicognia, J.M. Gili & R.G. Hughes).]
Soule, D.F. & Soule, J.D., 1979. Bryozoa (Ectoprocta). In Hart, C.W. & Fuller, S.L.H. (eds), Pollution ecology of estuarine invertebrates. New York: Academic Press, pp. 35-76.
Standing, J.D., 1976. Fouling community structure: effect of the hydroid Obelia dichotoma on larval recruitment. In Coelenterate ecology and behaviour (ed. G.O. Mackie), pp. 155-164. New York: Plenum Press.
Stebbing, A.R.D., 1971a. Growth of Flustra foliacea (Bryozoa). Marine Biology, 9, 267-273.
Stebbing, A.R.D., 1971b. The epizoic fauna of Flustra foliacea [Bryozoa]. Journal of the Marine Biological Association of the United Kingdom, 51, 283-300.
Suchanek, T.H., 1993. Oil impacts on marine invertebrate populations and communities. American Zoologist, 33, 510-523. DOI https://doi.org/10.1093/icb/33.6.510
Todd, C.D. & Turner, S.J., 1988. Ecology of intertidal and sublittoral cryptic epifaunal assemblages. II. Non-lethal overgrowth of encrusting bryozoans by colonial ascidians. Journal of Experimental Marine Biology and Ecology, 115, 113-126.
Veale, L.O., Hill, A.S., Hawkins, S.J. & Brand, A.R., 2000. Effects of long term physical disturbance by scallop fishing on subtidal epifaunal assemblages and habitats. Marine Biology, 137, 325-337.
Citation
This review can be cited as:
Last Updated: 17/08/2002